High-Temperature Fuel Cells for Mobile and Stationary Applications
Development of Intermediate-Temperature Fuel-Flexible Protonic-Ceramic Fuel Cells
This 5-year, $6.5M funded project is a part of the REBELS ARPA-E program and is focused on the development of intermediate-temperature, protonic ceramic fuel cell (PCFC) technology. The realization of an intermediate-temperature (500-600°C), high-efficiency direct-reforming hydrocarbon-fueled fuel cell system is an unquestioned “holy grail” in the fuel cell community. Unfortunately, the commercialization of conventional, direct-hydrocarbon fuel cells based on solid-oxide fuel cell (SOFC) technology has historically been impeded by a number of challenges associated with high operating temperatures (800-1000°C), degradation and coking issues, and complex and expensive cell manufacturing. The PCFC technology being developed at Mines is well suited to fix the shortfalls of the current SOFC technology because the fuel cells can operate at lower temperatures, are not subject to fuel dilution at high fuel utilization like SOFC’s (see Figure 1), and have the potential to be manufactured at a lower cost. In collaboration with the CSM Profs. Robert J. Kee, Sandrine Ricote, Ryan O’Hayre and Neal Sullivan and Fuel Cell Energy, our team is developing a mixed proton (H+) and oxygen ion (O2-) conducting electrolyte that allows a fuel cell to operate at temperatures less than 600°C, which is a significant departure from today’s ceramic fuel cells. The benefits of the technology are fuel cell stack cost reductions of 25-40% compared with SOFCs [1], fuel flexibility to operate directly on liquid and gaseous hydrocarbons [2,3], and electric efficiencies that could reach up to 70%-LHV without hybridization [4]. Recent technology scale up efforts at Mines and Fuel Cell Energy have resulted in the fabrication of commercial sized BCZYYb-based cells that maintain the high performance of the button cells produced at Mines [5]. A multi-scale modeling approach is pursued by the AESG team. Small scale models at the cell level (~micrometer) capture the performance of the fuel cell across a wide range of operating conditions. Larger scale stack models (~centimeter), groups of individual cells wired in series, are used to increase the amount of power produced by the fuel cells such that the total power produced is of useful quantity. System models (~meter) capture heat recuperation and necessary balance-of-plant operations that ensure the fuel cell system is operated properly as a stand-alone power generator. Lastly, techno-economic analysis (TEA) is used to aid in system design, technical, and economic performance over the lifetime of the system.
The development of a new protonic ceramic fuel cell computational modeling framework is presented for a predictive cell-level, interface charge transfer PCFC model capturing the mixed conducting nature of the protonic ceramic materials [6,7]. The model employs a 1-D channel- level modeling strategy where fuel depletion and flow configuration effects are resolved and coupled to a semi-empirical electrochemical model. Modeling results calibrated against experimental data of a state-of-the-art PCFC composition are presented. The model is capable of simulating a fuel flexible PCFC where methane and steam, without pre-reforming, are fed directly into the anode gas channel. Figure 2 depicts the cell modeling space, and shows a small scale PCFC stack. The goal in modeling the PCFC is to characterize fuel cell performance across a wide range of operating conditions so that the fuel cell/stack sub-model can be integrated into a complete fuel cell system model. The fuel cell system is designed around operating constraints of the fuel cell such as reactant temperature, fuel composition, cell temperature, cell operating voltage, and cell temperature gradients so that the cells maintain their performance over the lifetime. The PCFC system is designed to operate using a standard natural gas fuel source with the objective of providing quiet, reliable, high efficiency distributed electricity to the consumer.
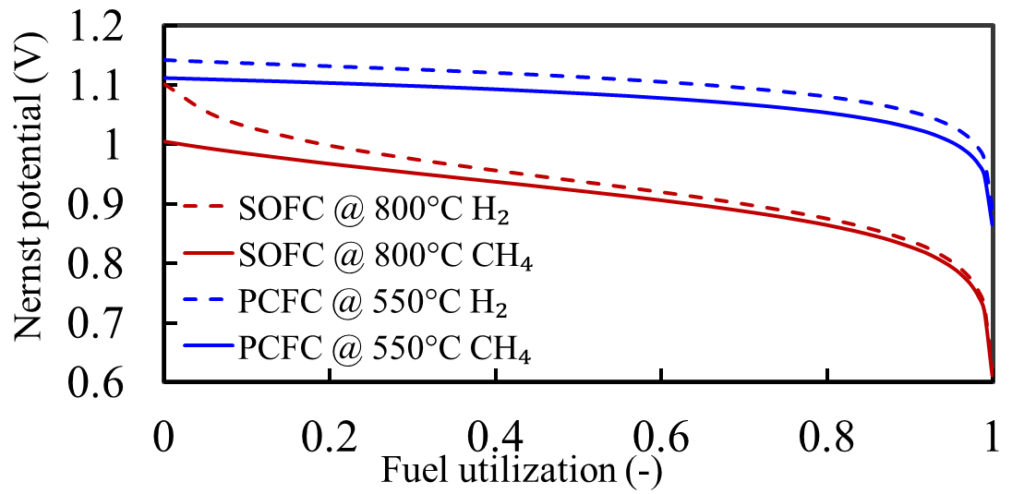
Figure 1: PCFC operation enables higher theoretical fuel utilizations than SOFC. SOFC’s produce steam in the anode as the reaction progresses, this dilutes the fuel and lowers chemical driving force. PCFC’s primarily produce steam in the cathode, so they do not suffer from this problem.
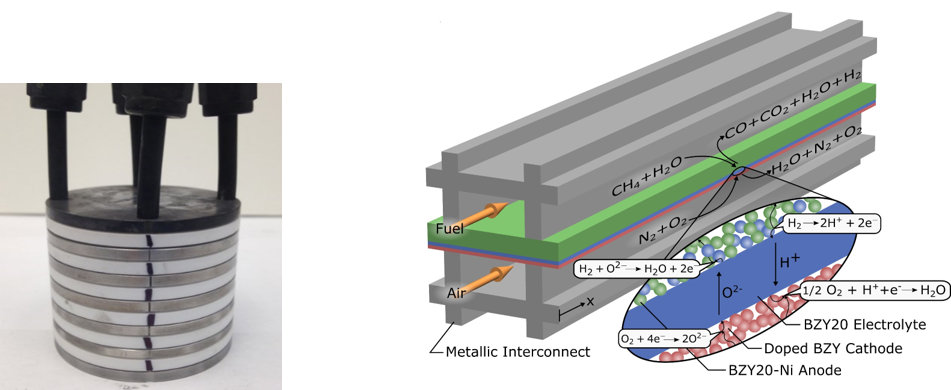
Figure 2: A 6 cell lab-scale prototype PCFC stack (left). A schematic depicting some of the key reactions occurring in the PCFC cell (right).
Recent progress in the performance of intermediate temperature (500-600°C) protonic ceramic fuel cells (PCFCs) has demonstrated both fuel flexibility and increasing power density that approach commercial application requirements. At this point in the technology maturation, it is critical to perform TEA in order to project the cost of the technology. The recent developments may eventually position the technology as a viable alternative to solid oxide fuel cells (SOFCs) and molten carbonate fuel cells (MCFCs). The PCFCs investigated in this work are based on a BaZr0.8Y0.2O3-δ (BZY20) thin electrolyte supported by BZY20/Ni porous anodes, and a triple conducting cathode material comprised of BaCo0.4Fe0.4Zr0.1Y0.1O3-δ (BCFZY0.1). These cells are prepared using a low-cost solid-state reactive sintering (SSRS) process, and are capable of power densities of 0.156 W cm-2 at 500°C operating directly from methane fuel. We develop a manufacturing cost model to estimate the Nth generation production costs of PCFC stack technology using high volume manufacturing processes and compare them to the state-of-the-art in SOFC technology1. The low-cost cell manufacturing enabled by the SSRS technique compensates for the lower PCFC power density and the trade-off between operating temperature and efficiency enables the use of lower-cost stainless steel materials. PCFC stack production cost estimates are found to be as much as 27-37% lower at 550°C than SOFCs operating at 800°C (see Figures 3 and 4).
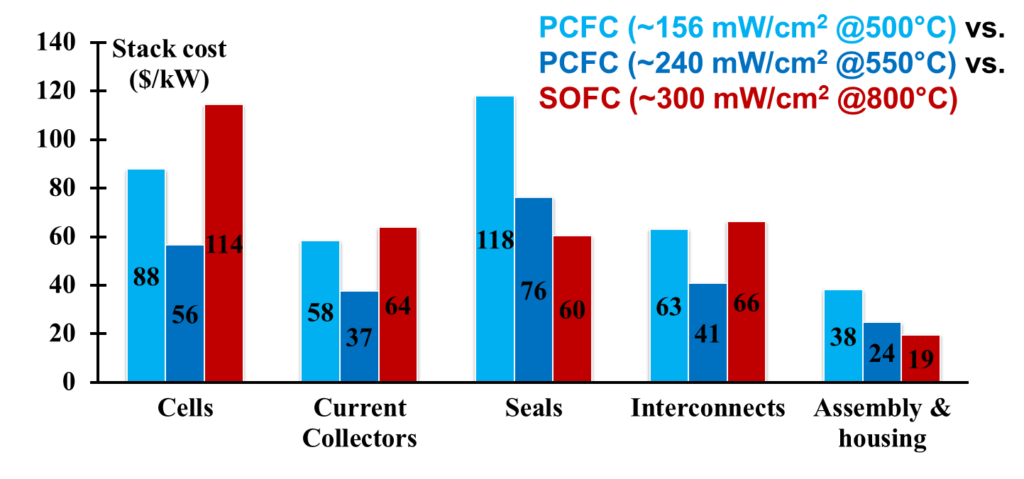
Figure 3: Lower grade stainless steel enables cost reduction for current collector & interconnect.
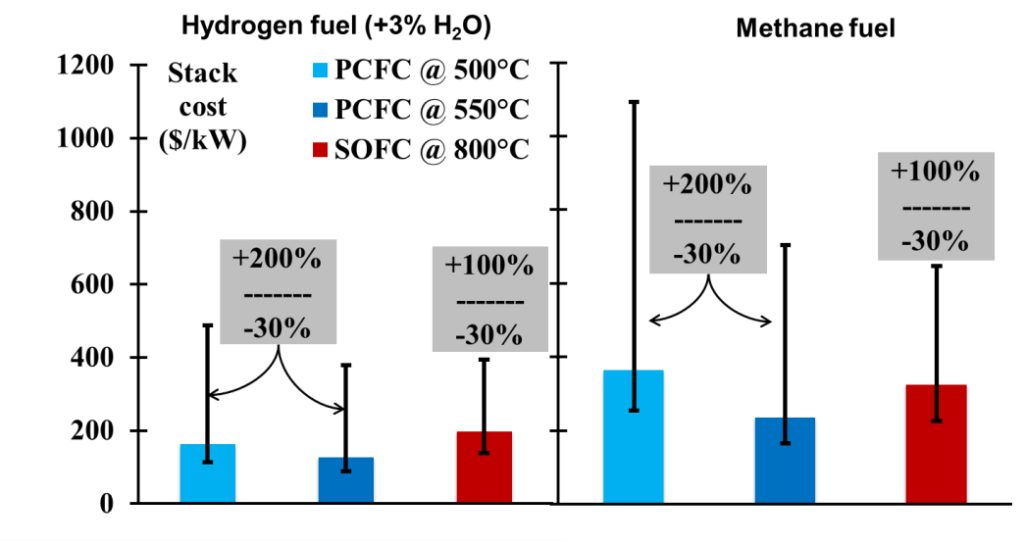
Figure 4: Cost analysis uncertainty still projects competitive PCFC vs. SOFC.
The capital cost of the complete system is determined by estimating the cost of each of the individual balance-of-plant (BOP) components. The stack cost is estimated using the model above since this technology is new and there are no cost correlations. The other BOP equipment, like heat exchangers and blowers, are well established, so correlations are used to determine their capital cost. Instrumentation, controls, and other minor pieces are estimated as a function of system size and compared to other fuel cell technologies. The REBELS project aims to position the PCFC system as a small-scale distributed generation (DG) prime mover because it can operate at high efficiency and be low cost even at the kW level. The system TEA model is run for 10 kW net AC power and 25 kW net AC power so that differences in cost due to varied system size can be investigated. Simultaneously, the steady state performance and cost of the system are solved.
FUNDING: DOE ARPA-E Program
PUBLICATIONS
- A. Dubois, K. Ferguson, R.J. Braun, Design of Protonic Ceramic Fuel Cell Systems and Their Potential as a Distributed Power Generator with Electric Efficiencies Exceeding 70%, working paper for Journal of Power Sources (2020).
- R.J. Braun, A. Dubois, K. Ferguson, C. Duan, C. Karakaya, R.J. Kee, … & A. Wood, Development of kW-Scale Protonic Ceramic Fuel Cells and Systems. ECS Transactions, 91(1):997–1008, (2019).
- K.J. Albrecht, A. Dubois, K. Ferguson, C. Duan, R.P. O’Hayre, R.J. Braun, Steady-State and Dynamic Modeling of Intermediate-Temperature Protonic Ceramic Fuel Cells, Journal of the Electrochemical Society, 166(10), F687–F700, (2019).
- C. Duan, S. Ricote, H. Zhu, N. Sullivan, C. Karakaya, R.J. Kee, R.J. Braun, R. O’Hayre, Highly Durable, Coking and Sulfur Tolerant, Fuel-Flexible Protonic Ceramic Fuel Cell, Nature, 557:217–222, (2018).
- A. Dubois, S. Ricote, R.J. Braun, Benchmarking the Expected Stack Manufacturing Cost of Next Generation, Intermediate-Temperature Protonic Ceramic Fuel Cells with Solid Oxide Fuel Cell Technology, Journal of Power Sources, 369:65–77, (2017).
- C. Duan, J. Tong, M. Shang, S. Nikodemski, M. Sanders, S. Ricote, A. Almansoori, R. O’Hayre, Readily Processed Protonic Ceramic Fuel Cells with High Performance at Low Temperatures, Science, 349(6254):1321–1326 (2015).
- K.J. Albrecht, C. Duan, R. O’Hayre, R.J. Braun, Modeling Intermediate Temperature Protonic Ceramic Fuel Cells, ECS Transactions, 68(1):3165–3175, (2015).
Current Projects
Past Projects
Selected Publications in This Research Area
Design of Protonic Ceramic Fuel Cell Systems and Their Potential as a Distributed Power Generator with Electric Efficiencies Exceeding 70% (working paper)
A. Dubois, K. Ferguson, R.J. Braun
Journal of Power Sources, (2020)
Development of kW-Scale Protonic Ceramic Fuel Cells and Systems
R.J. Braun, A. Dubois, K. Ferguson, C. Duan, C. Karakaya, R.J. Kee, … A. Wood
ECS Transactions, 91(1):997–1008, (2019)
Steady-State and Dynamic Modeling of Intermediate-Temperature Protonic Ceramic Fuel Cells
K.J. Albrecht, A. Dubois, K. Ferguson, C. Duan, R.P. O’Hayre, R.J. Braun
Journal of The Electrochemical Society, 166(10):F687–F700, (2019)
R.J. Braun, E. Reznicek, C. Cadigan, N.P. Sullivan, R. Danforth, T. Bandhauer, S. Garland, D. Olsen, B. Windom, B. Schaffer
ECS Transactions, 91(1):355–360, (2019)
Highly Durable, Coking and Sulfur Tolerant, Fuel-Flexible Protonic Ceramic Fuel Cell
C. Duan, S. Ricote, H. Zhu, N. Sullivan, C. Karakaya, R.J. Kee, R.J. Braun, R. O’Hayre
Nature, 557:217–222, (2018)
G. Anyenya, R.J. Braun, K. Lee, N.P. Sullivan, A. M. Newman
Optimization & Engineering, 19:1037–1081, (2018)
A. Dubois, S. Ricote, R.J. Braun
Journal of Power Sources, 369:65–77, (2017)
G.Anyenya, N.P. Sullivan, R.J. Braun
Energy Conversion & Management, 140:247–259, (2017)
G.Anyenya, B. Haun, M. Daubenspeck, R. J. Braun, N.P. Sullivan
ASME Journal of Electrochemical Energy Conversion & Storage, 13(4):041001, (2016)
Readily Processed Protonic Ceramic Fuel Cells with High Performance at Low Temperatures
C. Duan, J. Tong, M. Shang, S. Nikodemski, M. Sanders, S. Ricote, A. Almansoori, R. O'Hayre
Science, 349(6254):1321–1326, (2015)
Modeling Intermediate Temperature Protonic Ceramic Fuel Cells
K.J. Albrecht, C. Duan, R. O’Hayre, R.J. Braun
ECS Transactions, 68(1):3165–3175, (2015)
K. Pruitt, A. Newman, S. Leyffer, R.J. Braun
Optimization and Engineering, 15:167–197, (2014)
K. Pruitt, R.J. Braun, A. Newman
Applied Energy, 102:386–398, (2013)
K. Pruitt, R.J. Braun, A. Newman
Applied Energy, 111:904–920, (2013)
Application of SOFCs in Combined Heating, Cooling and Power Systems
R.J. Braun and P. Kazempoor
Chap. 12 in Solid Oxide Fuel Cells: From Materials to System Modeling, T.S. Zhao and M. Ni, editors, Energy and Environment Series No. 7, Royal Society of Chemistry, Cambridge, U.K. (2013)
View Other Research Areas:
MODELING AND SYSTEMS ANALYSIS OF ALTERNATIVE FUEL PRODUCTION AND UTILIZATION SYSTEMS
RENEWABLES AND GRID-ENERGY STORAGE SYSTEMS